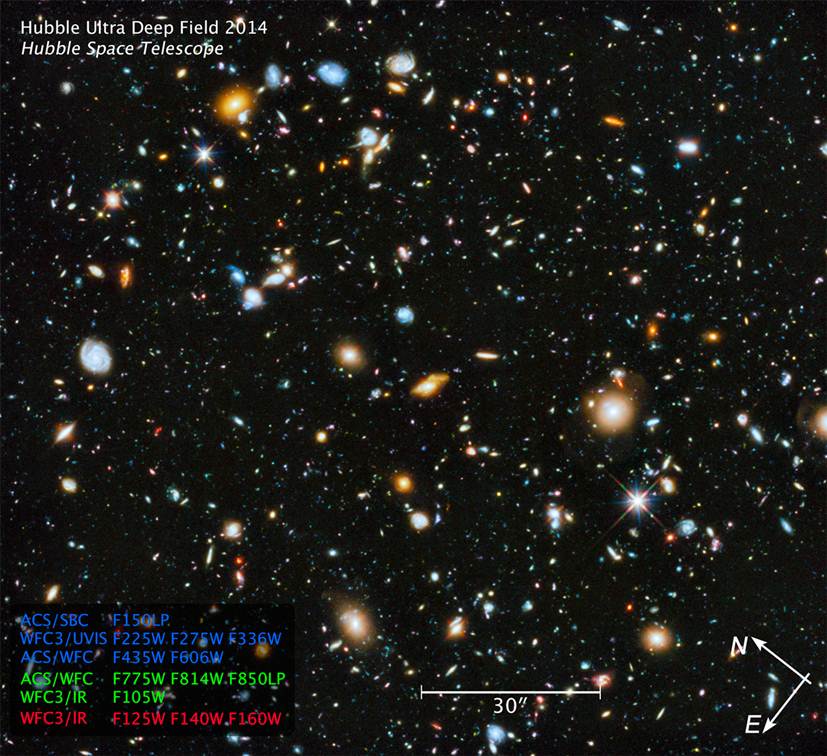
By Charlie Wood
It was the scientific equivalent of LeBron James, Steph Curry and Kevin Durant running a college basketball camp together: For two weeks in late July, three giants of string theory joined other leading physicists at Princeton’s Institute for Advanced Study to give a series of talks. The goal of Edward Witten, Juan Malcedena, Leonard Susskind and their colleagues was not to share their latest results nor necessarily to brainstorm new ones. Rather, they were holding a summer school to educate grad students and other early career physicists about what could be the next big idea in theoretical physics.
They ran it hard, recalls Geoff Penington, a participant from Stanford University. “You arrived at 8:45 for breakfast, left at 8 p.m. after dinner, and all the time between was lectures. Sometimes you got so exhausted, you slept in and skipped.”
The payoff for all that hard work? An entirely new way to think about the world. Physicists have spent centuries developing a precise accounting of energy and mass, a bean counting of joules and kilograms that has been a smashing success in describing everything from apples to sunbeams. But now some theorists are wondering if that approach could be backwards.
It’s not about stuff. It’s about information — not unlike the files that pile up on your hard drive and the pixels you stream from Netflix. Or at least that’s the key idea driving both the summer school, From Qubits to Spacetime, and a research program moving along similar lines, It from Qubit.
The shift is a radical one. We generally think of information as something generated by our activities, like tweeting, posting on Instagram or writing a novel, but that perspective might not be the only one. Rather, information could be responsible for driving the drama of the evolving universe, and — wait for it — even stitching together the fabric of reality itself. In some sense, the proper way to measure the space in front of you could be with bytes and bits, not feet and inches. And in addition to changing how we think about space, the new perspective could even help solve the top problem in modern physics — figuring out how gravity works on the particle level.
But making that admittedly bonkers-sounding case relies on an underappreciated area of math that not even physicists necessarily learn in school. That’s why Edward Witten, the father of modern string theory, kicked off the summer program with a humble lecture addressing a simple-sounding question. What the heck is information anyway?
Fundamentally, information is an outcome from a limited menu of choices. The selection can be made by a human, such as when a proposal elicits a yes or a no, or by nature, such as when a coin toss decides who gets to eat the last chicken wing. Both of these cases, each with their two possible outcomes, represent the fundamental piece of information. Dubbed a “bit” in 1948 by mathematician Claude Shannon, this set of two alternatives forms the foundation of all data structures. Every text message, every picture and every movie can be reduced to a string of such yes-or-no choices.
With technology, we can see the tangible record of this binary. Every photo you’ve ever seen and every song you’ve ever streamed can be traced back to an array of tiny magnets either on your device or somewhere in a tech giant’s data center, each pointing up or down. A zero or a one. And with physics, we can see that this tangibility makes each bit a permanent presence in the universe.
Not even Shannon fully appreciated just how physical information was at first. A World War II codebreaker, he thought of information in the context of messages. His initial attempts to measure information related to the number of possible messages a given code could display. Consider a scheme employing the letters A, B and C, for example. How many messages could you write with just two characters? Making a list suggests six possible outcomes: AA, AB, AC, BB, CC, and BC. In this way, both message length and the number of possible characters come into play. The full equation, Shannon noted with interest, identically matched that of the physical notion of entropy, so he dubbed his quantity “information entropy.”
Often described as the ability of a system (such as a steam engine) to move stuff around, measuring entropy is also based on counting possible configurations. A steam engine works by boiling water and letting the expanding steam push outwards, but it only works if the steam has room to expand. In a bigger space, there are more ways to arrange the steam particles, so the entropy of the engine rises as the steam expands. This is similar to how the amount of information a message can hold increases as the message gets longer or as you start using emojis in addition to the alphabet. In both cases, entropy comes down to tallying up the possibilities.
In the early 1960s, physicists realized that this connection was no coincidence. Information and entropy, often expressed as heat, were two sides of the same coin. This conclusion made information as indestructible as a pound of gold. You can try to erase your hard drive, but all that information just gets carried off as heat — inaccessible, but no less real.
Witten’s introductory lecture back at the summer camp quickly diverged from this so-called classical theory of information, however, because modern physics tells us that reality isn’t classical. It’s quantum. Just as matter can’t be made up of discrete pixels, neither can information. Quantum information replaces yes-or-no bit with something called a “qubit,” which is more of a yes and a no. If a bit represents a selection between a yes and a no, qubits represent a selection between a yes, a no, and everything in between (including half a yes and half a no, and all the other yes-to-no ratios you can imagine).
In a computer, if a magnet pointing up or down represents one bit, you can think of a qubit as the information held in an electron pointing in many directions at once. Thus the continuous qubit replaced the binary bit as the universe’s ultimate carrier of information and the object of physicists’ affections. Wherever particles go, they realized, qubits go too.
But before we can understand how these information carriers might actually be space-time Legos, we need to take a look at something entirely familiar yet deeply mysterious: the force that keeps our feet on the ground.
The top question in theoretical physics, and the top objective of the It from Qubit collaboration, is the quantum nature of gravity. A quantum is a particle — which you can alternatively describe as a ripple moving through space — and the universe seems to be full of them. In practice, physicists can describe almost any event imaginable with this ripple framework — except for happenings that involve gravity.
That force alone gets a separate, particle/ripple-free geometrical description from Einstein’s theory of general relativity: Objects bend space, which in turn bends the path of other objects. That story works fine for planets and apples, but not for wavelike photons and electrons. If a particle is spread out as a wave, what does the warping of space from that wave look like? No one knows, but most physicists feel that the question should have an answer.
One attempt at finding such an answer has gained a perhaps surprising degree of popularity, considering how insane it might sound at first: The universe could be more of a flat hologram (like the kind on your credit card) than it is a 3-D space. Garnering more than 16,000 citations since 1997, the paper describing this theory, courtesy of Princeton physicist Juan Malcedena, gives the gravity problem more of a workaround rather than a solution. Drawing on well-known results related to black hole surface area and volume, he demonstrated a sort of equivalence between the main part of a universe (called simply “the bulk”) and the flat surface that wraps around it (called “the boundary”).
The bulk is a simpler version of our messy universe with a different shape. The so-called “duality” between the bulk and its boundary means that for any situation in the middle, like a beam of light traveling through empty space, there is a perfectly accurate description of that situation on the boundary.
Renowned string theorist Leonard Susskind often likens the situation to an aluminum can: We would live in a 3-D space in the middle of the can, yet somehow, the flat label has enough information to perfectly describe anything that goes on inside. We experience something similar when we look at the shiny hologram on a credit card. It has enough information to describe a 3-D object because it looks like a 3-D object, yet it’s printed on a flat sticker.
But gravity, which no one understands on a microscopic level, exists only inside the can in this scheme, not on the label. Despite not knowing how a particle’s wave bends space, physicists can mathematically convert that can situation into the corresponding label description and use run-of-the-mill physics there to get a prediction that holds true in both places. The process is something like working out a “trains leaving two cities” problem out in kilometers because the math is easier, and then changing your answer back into miles at the end.
On the boundary, “we know all the rules of the game,” says University of Maryland physicist Brian Swingle. “On the gravity side, we don’t even know all the rules.”
Despite the popularity of these mathematical games some researchers outside of the It from Qubit collaboration wonder if they’ll ever yield real answers about how gravity works in the real universe.
“To be honest, I’m not totally convinced yet how much it will go beyond the translation,” says Sergei Dubovsky, a physicist at New York University who uses string theory tools to study the strong force. “It’s one thing to phrase the same problems in different languages, but really one wants more.”
But if the bulk-boundary translation does provide lasting insight, what does this correspondence have to do with information? The last piece of the puzzle came from a provocative 2006 paper from Japanese researchers Shinsei Ryu and Tadashi Takayanagi examining an odd quantum phenomenon called entanglement — two particles forming one system that stays connected even when separated. They showed that when those particles/qubits live on the boundary, entropy ties them together with the path that crosses the bulk. In other words, physicists can use entropy, a measure of information, to figure out the shape of the bulk based on the arrangement of particles on the boundary, and vice versa, Swingle says.
Subsequent research on the new bulk-boundary game has probed the three-way relationship between entropy, entanglement and the aluminum can picture to address the question posed by the names of both the It from Qubit collaboration and the Spacetime from Qubit summer school. Could the space of the bulk somehow be made of information?
Ask a mind-bending question to a string theorist, and you’ll get a mind-bending answer. Swingle is one of a number of physicists compelled by the notion that space itself is what physicists call “emergent,” something that seems real enough to our senses but actually springs from the collective behavior of tiny objects. Eighteenth century scientists, for example, thought that heat was a fluid, but we now understand that it’s just the energetic motion of particles. Emergent theories of space seek a similarly basic description, with entangled pairs emerging as one strong candidate for a possible building block.
“Entanglement on the boundary is what causes the bulk to be connected,” Penington, the Stanford University participant, explains.
The situation vaguely resembles a circular embroidery pattern. Connect all the points on the edge of the circle with each other, and eventually you’ll have woven a cloth that covers the entire middle area. It’s kind of like that, except the edge is the boundary, the middle area is the bulk, the points on the edge are particles, the threads are entanglement and the pattern is devilishly complicated.
Let’s take a step back and recap that madness. Entangled particles on the boundary draw “threads” across the bulk that knit together what you and I would call space. The shape of that space depends on how much entanglement you have between qubits/particles on the boundary. Finally, the work of Ryu and Takayanagi suggests that entanglement is wrapped up with entropy, which is really information. Or so It from Qubit story goes.
This new picture of reality as an information byproduct is a far from proven, or even all that useful. It describes a universe much simpler than ours, and the mathematics of the aluminum can duality haven’t been rigorously proven. But where all this pie-in-the-sky theorizing comes down to Earth is its occasional ability to make new predictions that were previously impossible. “We’ve made a lot of progress,” Swingle says, “to the point where we even understand in certain simple cases how to decode the information that falls into a black hole.” Should those calculations hold up, some theorists hope the bulk-boundary duality will lead the way toward the ultimate reward, an end to the century-long quest for a quantum description of gravity.
Top Image Credit: NASA, Hubble Space Telescope